Cellular and Bacterial Genetic Sequences in
Monkey-Derived Stealth Adapted Viruses by W John Martin in Cohesive Journal of Microbiology & Infectious Disease_open infectious diseases journal impact factor
Abstract
Stealth adapted viruses differ from the viruses from which they are
derived in not being effectively recognized by the cellular immune
system. This is because of the deletion or mutation of the genes coding
for the relatively few virus components, which are generally targeted by
cytotoxic T lymphocytes. Stealth adapted viruses do not, therefore,
normally evoke inflammation, the hallmark of most infectious illnesses. A
stealth adapted virus was repeatedly cultured from the blood of a
patient with the chronic fatigue syndrome (CFS). Polymerase chain
reaction (PCR) performed on the culture identified the virus as being
derived from an African green monkey simian cytomegalovirus (SCMV). The
PCR also amplified a genetic sequence closely related to a normal
cellular gene. Further analysis of the viral DNA indicated that it was
fragmented and genetically unstable. Moreover, additional genetic
sequences have been incorporated into the replicating virus genome.
Several of the additional sequences are originally of cellular origin
with subsequent genetic modifications. Other incorporated sequences are
of bacteria origin. PCR performed on cultures from some other CFS
patients, led only to the amplification of modified cellular sequences,
including sequences clearly derived from the rhesus monkey genome. It is
proposed that as part of the stealth adaptation process, sequences of
the original infecting virus can be largely displaced by cellular and/or
bacteria sequences, which have essentially switched their affiliation
to that of the stealth adapted virus. For this reason, they are referred
to as renegade sequences. The term “renegade viruses.” is also proposed
to describe those viruses in which the originating conventional virus
sequences have yet to be detected. The findings are relevant to efforts
to seek a virus cause of many common illnesses, including CFS, and to
the possible misattribution of certain illnesses to bacterial
infections.
Keywords: Renegade viruses; Stealth adapted viruses;
Chronic fatigue syndrome, Polio vaccine; noncoding RNA; Ochrobactrum;
Mycoplasma; Cytomegalovirus; Viteria
Abbreviations: ACE: Alternative Cellular Energy;
CFS: Chronic Fatigue Syndrome; CPE: Cytopathic Effect; CFS:
Cerebrospinal Fluid; NCBI: National Center for Biotechnology
Information; NT: Nucleotide; PCR: Polymerase Chain Reaction; RhCM:
Rhesus Monkey Cytomegalovirus; SCMV: African Green Monkey Simian Cytomegalovirus
Introduction
A stealth adapted virus was repeatedly cultured from a CFS patient
[1]. It induces a foamy vacuolated cytopathic effect (CPE) when cultured
on human and animal cells, including human foreskin fibroblast cells
(MHRF). Electron microscopy of the infected cells indicate the presence
of both herpesvirus-like particles and intracellular inclusions. The
polymerase chain reaction (PCR) was employed to identify the type of
virus infecting the cells. Among the sets of primers used in the PCR
were a forward primer “SK43” (5’-cggatacccagtctacgtgt-3’), which matches
to sequence within the tax gene of human T lymphotropic virus-1
(HTLV-I) and a backward primer “SK44” (5’-gagctgacaacgcgtccatcg-3’),
which matches to a sequence within the tax gene of HTLV-II [2].
Using relatively low stringency PCR, this set of primers yielded
several PCR products when performed on the infected cultures, with no
identifiable products being formed when using uninfected cultures [1].
The PCR products were cloned and sequenced. A radiolabeled PCR product
was also used to identify virus DNA in extracts of infected cells and in
the pelleted material obtained by ultracentrifugation of filtered
culture supernatant. In agarose gel electrophoresis, the virus DNA in
the pelleted material migrated with an estimated size of approximately
20 kilobases (kb) [1].
Cytomegalovirus-related DNA sequences
The PCR assay yielded prominent bands in agarose gel electrophoresis
of approximately 0.5 and 1.5kb [1]. The 1.5kb band was subsequently
shown to contain two PCR products, measuring 1,487 and 1,510
nucleotides, respectively. The PCR amplified sequences were flanked at
both ends by the SK44 primer. Using the BLASTN and BLASTX programs [3],
the amplified sequences were compared with the then available genetic
sequences on GenBank; a repository of nucleic acid and protein sequences
maintained by the National Center of Biotechnology Information (NCBI).
The analysis showed that the longest sequence (included in NCBI
Accession number U09212) was related to but still different from a
sequence within human cytomegalovirus. As more GenBank sequences became
available for comparison, the sequence of this PCR product was shown to
have far greater sequence homology with African green monkey simian
cytomegalovirus (SCMV) [4]. Similarly, the other large PCR product (NCBI
Accession number U09213) was subsequently shown to match closely to a
sequence within SCMV with 1348/1454 (93%) identical nucleotides, with 19
gaps [5]. The next closest match of the sequence of the PCR product is
to the cytomegalovirus of Mandrillus (Dril) monkey with 938/1389 (68%)
identical nucleotides and 124 gaps. There was even less overlap with the
best matching rhesus monkey cytomegalovirus (RhCMV,) which had 364/515
identical nucleotides. Using the standard BLASTN program, there was no
significant matching of the sequence in U09213 with human
cytomegalovirus.
Detection of cellular-derived DNA sequence
The smaller product that was amplified in the PCR on infected but not
on uninfected cultures was sequenced (NCBI accession number AF107851.1).
This product was flanked at both ends with primers in which there was a
single nucleotide difference (cytosine rather than adenosine at the 10th
position) than in the originally intended SK43 primer. The DNA sequence
of the PCR amplified product, excluding the primers, was analyzed
against the most recent “assembled” complete human genome, This showed a
near identical homology with an intergenic region of the human X
chromosome (NCBI accession number NC_000023.11), extending from
nucleotide 30,177,214 to nucleotide 30,177,822. Within this region there
were 606/609 identical nucleotides (99.51 percent identity) with 2
single nucleotide deletions and one nucleotide substitution (Figure 1).
When using the nr/nt (protein and nucleotide) database in the BLASTN
program, there were only two matches to the human genome (NCBI Accession
numbers AC117518.3 and AC005145.1). The next 3 matches, all with 97.5%
identity, were to a sequence in the chimpanzee genome. It was considered
possible that the X-chromosome-related sequence may have originated in
an African green monkey. On further analysis using the primates’
“assembled genomes,” there was decreasing overall relatedness of the PCR
product with the human genome to the genomes of chimpanzee, rhesus, and
Cercopithecus (African green) monkeys. The data are included in Table 1
and establishes the X-chromosome related genetic sequence as being
human in origin.
Figure 1: Matching of the sequence of the PCR product with that of a region of the human X chromosome. What
is shown is the BLASTN readout obtained by submitting to GenBank, the nucleotide sequence of a cloned PCR
product generated using the SK44/SK43 primers on DNA from the stealth adapted virus infected cultures. The
submitted sequence is labeled as the “Query.” The “Sbjct” is the nucleotide sequence of the subject item, which
is selected by the BLASTN program as having a matching nucleotide sequence. The vertical lines between the
Query and Sbjct indicate nucleotide identity. Gaps occur in regions where one or more nucleotides are in only
one of the pair of sequences. The numbers refer to numbered nucleotides in the Query and Sbjct. The Sbjct in
this Figure is the human X chromosome (NCBI accession number NC_000023.11).

Table 1: The relative numbers and percentages of identical nucleotides of the cloned PCR product shown in Figure 1
with matching sequences of humans and several primates.

Cloning of the virus genome
Filtered supernatant of infected cultures was ultracentrifuged as a
means of pelleting extracellular virus particles. DNA was extracted from
the pelleted material. In a second study, the extracted DNA was further
purified by electrophoresis into agarose gel, with excision of the
narrowly banded DNA. The DNA from the first study was cut using the
EcoR1 restriction enzyme and cloned into pBluescript plasmids (3B
series). The DNA in the second study was cut using the Sac1 restriction
enzyme and was also cloned into pBluescript plasmids (C16 series). At
least partial DNA sequence data were obtained from over 450 clones using
the T3 and T7 promoter sites on the pBluescript plasmid [5-7]. Several
of the more interesting clones were completely sequenced. The sequence
data on the individual clones were provided to GenBank under the listing
of stealth virus 1. Using the BLASTN and BLASTX programs of the NCBI,
the sequences fell into three categories. The majority of the clones
contained sequences, which matched reasonably closely to regions of the
SCMV genome. Several of the clones with sequences that match to the SCMV
genome have been assembled into longer stretches of non-overlapping
sequences. The NCBI accession numbers of these longer stretches are
listed in Table 2 and collectively they comprise 97,780 nucleotides. The
overall regions of the SCMV genome (length 226,205 nucleotides) which
the BLASTN program matches to the stretches of the stealth adapted virus
sequences are shown in Table 2, along with the number of identical
nucleotides. With sequences, U27627.2 and U27770.2, there was an
intervening region in which the stealth virus and SCMV sequences did not
adequately match. There are disproportionate numbers of clones, which
match to certain regions of the SCMV while there is no matching to other
regions. There is also a diversity in the sequences in some of the
clones, which match to the same region of the SCMV genome. These
differences include nucleotide substitutions, deletions, repetitions,
and recombination. Nevertheless, the results are unequivocal in
indicating that SCMV was the originating virus and that it has undergone
substantial genetic changes.
Table 2: BLASTN matching of the cloned sequences of stealth virus-1 with the sequence of Cercopithecine herpesvirus
5 strain 2715, NCBI FJ483968.2.

Detection of additional cellular sequences related to the human genome
A second category of cloned sequences were those that could be
matched to various specific regions of the human genome [8,9]. The
matching cellular sequences are in different human chromosomes. While
the sequences of some of the clones are identical to the matching region
of the human genome, many of the clones show minor differences. The
most common nucleotide changes are multiple inserts, deletions and
substitutions of single nucleotides. Occasionally longer inserts and
larger deletions are present. The frequency of inserted, deleted, or
substituted nucleotides in the clones, compared with the best matching
human sequence is quite variable, but commonly in the range of 2-4%.
Figure 2 shows portions of the BLASTN readouts obtained by submitting to
GenBank, the DNA sequences of three clones derived from the virus
culture. With each clone, the best matching subject sequences in
GenBank’s nr/nt database are different human cellular genes. It was also
thought possible that some of the clones might possibly match better to
the genome of African green monkeys. The sequence analysis was,
therefore, occasionally repeated using the assembled genome of African
green monkey. In no case so far examined, however, is the matching to
the monkey genome been closer than that to the human genome. Another
observation is that the regions of partial homology between the cloned
sequence and that of a human cellular gene is invariably occurring
within the non-coding region of the human genes. A more comprehensive
analysis of the relatedness of the cloned sequences to the human and
primate genomes is ongoing and will be provided in a subsequent
publication
Figure 2: Matching of cloned sequences with cellular sequences. What is shown are three examples in which a
region from both the T3 and the T7 partial readouts of the sequences of three clones obtained from the purified
DNA from the culture supernatant is directly compared with a matching Sbjct region of a cellular sequence. The
six selected cloned sequences are labeled as Query, whereas the Sbjct is best matching cellular sequence. The
numbers refer to the numbered nucleotides in the clones and in the Sbjct. The Sbjct for clones C16119 , C16128,
and C16261 are Homo sapiens chromosome 8 clone ABC9_45365100_H16, complete sequence AC275379.1;
Homo sapiens kazrin, periplakin interacting protein (KAZN), RefSeqGene on chromosome 1 Sequence ID:
NG_029844.2; and Homo sapiens isolate fa0190 immunoglobulin superfamily member 4B (IGSF4B) gene
AY663433.1, respectively.

Detection of bacteria-derived sequences
The third category of sequences identified in the cloned DNA are
clearly bacterial in origin [10]. Even at the initial time of submission
of the sequence data to GenBank, it was apparent that several of the
clones were very closely related to mycoplasma bacteria [11]. Examples
of clones with near-identical mycoplasma-related sequences are 3B35,
3B512, 3B528, and 3B632. The sequences these clones closely matched to
different sequences, which are essentially identical in plasmid 7 of
Mycoplasma conjunctivae strain NCTC10147 and in plasmid 9 of mycoplasma
fermentans strain M64 [12,13]. The numbers of identical nucleotides in
these four clones with the corresponding sequences in the mycoplasma
plasmids 2136/2142, 2342/2345, 2020/2044, and 1380/1384 respectively.
The sequences of several additional clones still preferentially match to
mycoplasma but with lower levels of nucleotide identity. An even larger
number of the clones have sequences, which at the time of submission to
GenBank, matched most closely to Brucella, an alphaproteobacterial
[14]. These sequences have subsequently been shown to have greater
homology to one or other species of Ochrobactrum [15]. Examples
of these clones are NCBI accession numbers 3B23, 3B41, 3B43, 3B47,
3B534, 3B614, C1616, and C16134.The different cloned sequences tend to
preferentially match to O. quorumnocens, with somewhat less matching to Ochrobactrum pituitosum and Ochrobactrum pseudogrignonense
[16-18]. Yet for some of the clones, there was only minimal or no
matching to O. quorumnocens. Some of these data are summarized in Table
3. For many of the cloned sequences, the changes from the best matching
Ochrobactrum species are rather minor with less than 1% nucleotide
substitution and only occasional gaps. Still other clones have
sequences, which, although seemingly of bacterial origin, cannot be
currently assigned to a species of bacteria. Some of these clones appear
to be broadly related to certain alphaproteobacteria, but only when
using the BLASTX program of the NCBI. An example is clone 3B513 (NCBI
accession number U27894.2), which comprises 8,106 nucleotides. Other
cloned sequences of apparent bacteria origin show a weak association
with Actinobacteria.
Table 3: The relative matching of the cloned sequences from the DNA of the stealth adapted virus cultures with three
species of Ochrobactrum bacteria.
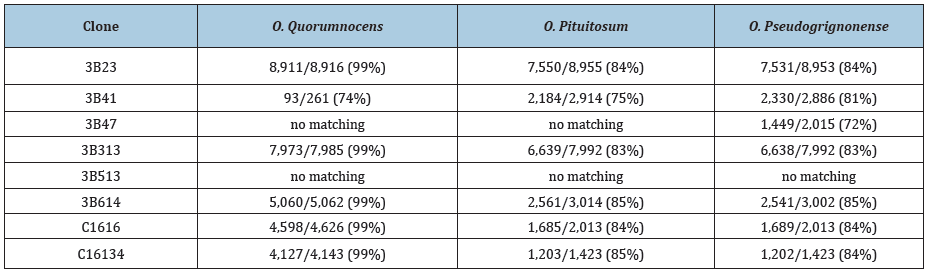
Genetically unstable and fragmented virus genome
As noted above, the sequences in many of the clones correspond to
diverse regions of the SCMV genome. There were many examples of
significant differences between clones even when they matched to the
same region of the SCMV genome. These differences were attributed to
genetic instability of the virus genome, including mutations, deletions,
insertions, and recombination. Furthermore, the aggregate length of
non-overlapping virus sequences far exceeded the estimated 20 kb genome
size based on the hybridization pattern seen in agarose gel
electrophoresis. This result is consistent with a fragmented genome
[19]. It is also possible that some of the DNA fragments are held
together via RNA, which can be subjected to breakage from environmental
sources of RNases.
PCR assays on other stealth adapted virus cultures
Figure 3: Matching of cloned sequences from culture A with a cellular sequence. What is shown is the BLASTN
readout of the nucleotide matching of the “Query” sequence of a cloned PCR product from culture A with a
sequence within Homo sapiens Rho guanine nucleotide exchange factor 10 (ARHGEF10) gene on chromosome
8 (NCBI accession number NG_008480.1). Note the 13-nucleotide insert into the cloned DNA sequence from
nucleotide 276 to 288.

The SK43/SK44 set of PCR primers was used in PCR assays on positive
stealth virus cultures obtained from several other patients. A strongly
positive culture was obtained from the cerebrospinal fluid (CSF) of a
comatose patient with a 4-year history of a bipolar psychosis [20]. The
culture gave similarly sized PCR products as did the previous culture.
Partial sequencing on one of the products from this culture confirmed a
close homology to that of the earlier sequenced PCR product from the
prototype SCMV-derived stealth adapted virus ((NCBI Accession number
U09213). There were still significant minor differences between the
sequences of the two viruses and with the corresponding region of SCMV.
Several sets of PCR primers were also developed based on sequences in
the cloned region of the prototype SCMV-derived stealth adapted virus.
These primers gave positive results when tested directly on the CSF
obtained from the comatose patient. A positive culture from the CSF of
another CFS patient, gave a negative PCR in direct testing using these
primers sets. Yet the culture did show a positive PCR for SCMV-related
sequences when the RNA from the culture was first transcribed into DNA
using reverse transcriptase PCR.
PCR assays on other stealth adapted virus cultures
The SK43/SK44 set of PCR primers was used in PCR assays on positive
stealth virus cultures obtained from several other patients. A strongly
positive culture was obtained from the cerebrospinal fluid (CSF) of a
comatose patient with a 4-year history of a bipolar psychosis [20]. The
culture gave similarly sized PCR products as did the previous culture.
Partial sequencing on one of the products from this culture confirmed a
close homology to that of the earlier sequenced PCR product from the
prototype SCMV-derived stealth adapted virus ((NCBI Accession number
U09213). There were still significant minor differences between the
sequences of the two viruses and with the corresponding region of SCMV.
Several sets of PCR primers were also developed based on sequences in
the cloned region of the prototype SCMV-derived stealth adapted virus.
These primers gave positive results when tested directly on the CSF
obtained from the comatose patient. A positive culture from the CSF of
another CFS patient, gave a negative PCR in direct testing using these
primers sets. Yet the culture did show a positive PCR for SCMV-related
sequences when the RNA from the culture was first transcribed into DNA
using reverse transcriptase PCR. SK43/SK44 primers are sometimes able to
generate similarly sized, discrete PCR products when tested on positive
cultures from CFS patients. Three cultures yielded a product of
approximately 0.5kb, together with some smaller products. The larger
product from one of the cultures was cloned into pBluescript and fully
sequenced. Several PCR products from the other two cultures were
similarly cloned and partially sequenced from the T3 and T7 promotor
sites. The cultures were designated A, B, and C. The PCR product from
culture A (NCBI AF107850.1) was flanked on one end by the SK43 primer
and on the other end by the SK44 primer. The 507-nucleotide sequence
between the primers was compared with the nr/nt database on GenBank
using the BLASTN program. The top listed matching result was to Homo
sapiens Rho guanine nucleotide exchange factor 10 (ARHGEF10), RefSeqGene
(LRG_234) on chromosome 8 (NCBI accession number NG_008480.1).
The matching region extended from nucleotide 88302 to nucleotide 88794
of the cellular gene. This is a non-coding region of the gene located
between the 17th and 18th exons. There are 447/505
identical nucleotides (88.5%) with 16/505 gaps. A major gap occurs
because of a nucleotide stretch reading “acccccccccact” in the PCR
product between nucleotides 276 to 288. The matching is shown in Figure
3.
Rhesus monkey-related cellular sequences
The BLASTN program was used to further compare the sequence of the
PCR product with the sequences of the assembled human genome and that of
various primates. The results are presented in Table 4. The sequence of
the PCR product is clearly closer to the genome of the rhesus monkey
than to the human genome. There are 504/510 identical nucleotides
(98.8%) with 3/505 gaps. Moreover, the stretch of nucleotides in the PCR
product, which is absent in the human gene, is present along with two
additional cytosines in the rhesus genome. The matching of the PCR
product with the rhesus monkey genome is shown in Figure 4. Cloning of
the PCR products from the other two cultures, B and C, identified two
clones from culture B (C1113 and C1132). and one clone from culture C
(C1332), which all have sequences closely related to the rhesus
monkey-related sequence of the PCR product from culture A. These
comparisons are shown in Figure 5. Even when comparing the limited
sequences, the clones are slightly different from each other (Figure 5).
This even applies to the comparison of the sequences of the two related
clones obtained from culture B. Four additional clones obtained from
culture B and six additional clones obtained from culture C were also
partially sequenced using the T3 and T7 promotors on the pBluescript
plasmid. Even though the cultures were obtained from different patients,
there are two sets of matching clones. The T3 and the T7 sequences
beyond the primer sites of clone C1123 from culture B are like the
sequences read from the T7 and the T3 promotors in clone C1313 from
culture C (Figure 6). Similarly, the T3 and T7 readouts from clone C1142
from culture B are similar to the T3 and T7 readouts from clone C1335
from culture C (Figure 6). The remaining two clones from culture B and
four clones from culture C are different from one another. The two
clones (C1151 and C1163) from culture B matched better to the rhesus
monkey genome than to either the human or African green monkey genome.
The results with clone C1163 are shown in Figure 7. Yet three of the
remaining four clones from culture C show closer homology to the human
genome. An example with the T3 readout of clone C1311 is shown in Figure
8. The two other clones from culture C, which match more closely to the
human genome are clones C1333 and C1334.
Table 4: The relative number and percentages of identical nucleotides of the cloned PCR product from culture A with
matching sequences of humans and several primates.

Figure 4: Matching of cloned sequences from culture A with a rhesus monkey cellular sequence. What is shown
is the BLASTN readout of the nucleotide matching of the “Query” sequence of a cloned PCR product from culture
A with the Assembled genome of Macaca mulatta breed Indian rhesus macaque isolate RUp15 000818F, whole
genome shotgun sequence, SBKD01000790.1. There are 504 of 510 identical nucleotides with 3 gaps. This
matching is closer than that to the human cellular sequence as shown in Figure 3.

Figure 5: Alignments of the sequence of the cloned PCR product culture A with the sequences in cloned PCR
products from cultures B and C. What is shown are Query sequences from the T3 and T7 sequence readouts from
two clones from culture B (C1113 and C1132) and one clone from culture C (C1322) aligned with the sequence
of the PCR product cloned from culture A (Sbjct). The alignments did not include the SK43 and SK44 primers.
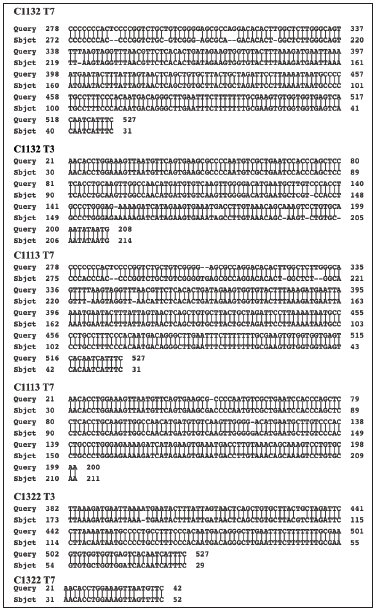
Figure 6: Alignment of matching clones from culture B and C. What is shown are the alignments between the
available T3 and T7 sequences of two sets of matching clones from culture B and culture C. These matching
clones were in addition to the clones which matched to the cloned PCR product from culture A. The alignments
show close similarity but not identity
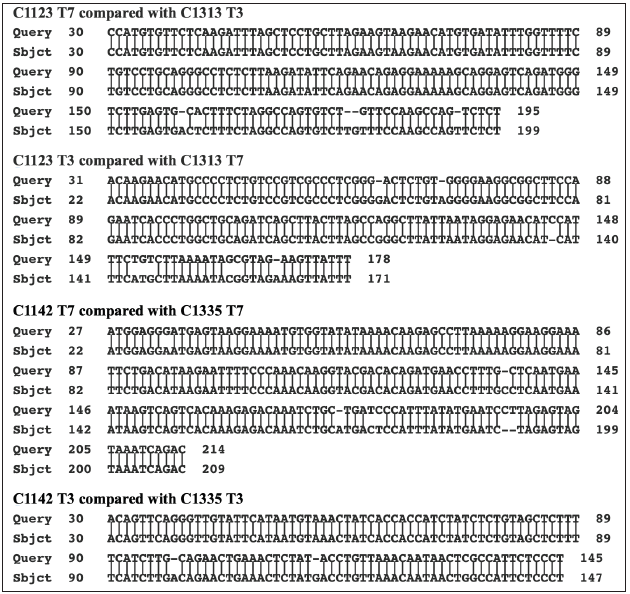
Figure 7: Matching of the sequence of the cloned PCR product with primate and human genomes. What is shown
is the sequence of clone C1162 from culture B as the Query, with the best matching cellular sequences (Sbjct)
in the rhesus monkey, African green monkey and human genomes. The chimpanzee genome gave the same
matching result as did the human genome.

Figure 8: Matching of the sequence of the cloned PCR product with rhesus monkey and human genomes What is
shown is the T3 sequence of clone C1311 from culture C as the Query, with the best matching cellular sequences
(Sbjct) in the assembled rhesus monkey, and human genomes. There were 235/275 (85%) identical nucleotides
with the rhesus monkey genome and 266/278 (96%) identical nucleotides with the human genome.

Discussion
These results provide additional information regarding stealth
adapted viruses. In addition to a mechanism for evading cellular
immunity, the process of stealth adaptation can apparently lead to
the substitution of some and potentially all of the originating virus
genome with other genetic sequences. The substituted sequences
can be cellular and bacterial in origin. These substituted sequences,
as well as any remaining initial virus sequences, can undergo further
changes due to the fragmentation and genetic instability of the
reformed viruses. The incorporation of both cellular and bacterial
sequences has occurred with an SCMV-derived stealth adapted
virus [6-10]. This virus is formally referred to as stealth virus-1. It
was repeatedly cultured from a CFS patient. Low stringency PCR
performed on virus infected cells using a fortuitous set of SK43/
SK44 PCR primers leads to the amplification of two long SCMVrelated
nucleotide sequences and a shorter sequence with near
identity to an intergenic region of the human X chromosome. No
discernable PCR products are generated when the PCR assay is
performed using the same set of primers on uninfected cells. The X
chromosome related sequence was amplified through loose crossreactions
with the SK43 primer, which inadvertently had a cytosine
rather than an adenosine as the tenth nucleotide. As was shown in
Figure 1, the amplified sequence differs from the matching region
in the human X chromosome in having two deleted nucleotides
and one nucleotide substitution. The effective amplification of the
sequence in the infected cells could possibly also be due to some
minor nucleotide changes which would allow for more effective
binding of the SK43 primer. This possibility can be addressed
by further sequencing of the primer binding sites of the virus
associated DNA. It is considered much more likely, however, that
the amplification seen with the virus infected cells reflects a greatly
increased copy number of the X chromosome-related sequence.
This is consistent with the X-chromosome-related sequence having
been incorporated into the virus replication process. Several
additional cellular derived sequences were identified upon cloning
of the DNA isolated from the supernatant of the stealth adapted
virus infected culture [6]. In one of the two cloning studies, the DNA had been further purified by agarose gel electrophoresis
prior to its restriction enzyme cutting and cloning into pBluescript
plasmids. This was intended to reduce the possible inadvertent
cloning of normal cellular DNA. Virus incorporated cellular DNA
can also be distinguished from normal cellular DNA if it has
undergone any mutations. Indeed, minor nucleotide differences
from normal cellular DNA were seen in many of the sequenced
clones with cellular-related DNA. As will be detailed in future
publications, the DNA in these and in other cellular sequencerelated
clones will typically match to intragenic, and occasionally
to intergenic, non-coding regions within a cellular genome. The
more common minor differences between the amplified sequences
and their matching cellular sequence are i) added or deleted single
nucleotides and ii) single nucleotide substitutions, each occurring
with an overall frequency of approximately 1%. The sequences in
several additional clones show far greater deviation from known
cellular or viral sequences, sometimes with no convincing matching
being obtainable to the human genome using the standard BLASTN
program. This lack of precise matching is in spite of the human and
many primate genomes having been fully sequenced. It is probably
a reflection of the genetic instability of the sequences, including
major insertions, deletions, substitutions, and recombination.
To date, there has been no decisive example of contiguous SCMV
and cellular sequences within the same clone. This type of
recombination is, however, fully anticipated to be seen in further
sequencing studies.
The prior incorporation of rhesus monkey cellular sequences
into stealth adapted viruses has occurred in the stealth adapted
viruses cultured in human cells from three CFS patients. These
cultures are designated A, B, and C. They were selected for further
study because in low stringency PCR assays using the SK43/SK44
primers, they yielded comparable patterns of PCR generated
products using. The cloned PCR product from culture A, two of
the cloned PCR products from culture B and one of the cloned PCR
products from culture C all match to the same region of the rhesus
monkey genome. As expected, the four PCR clones match to one
another, yet show individual differences. It could either be that the
three individuals were infected with the same variant strain of a
stealth adapted virus or that certain viruses have a propensity to
incorporate this particular rhesus cellular sequence. The finding
that cultures B and C have two sets of additional shared sequences
would tend to favor the first possibility. The sequence differences
between the matching clones from the different cultures, and
between the two matching clones from culture B, are consistent
with the genetic instability of stealth adapted viruses. The fact
that three of the seven cloned sequences from culture C match
better to a human sequence is consistent with homologous genetic
recombination. This could explain why the X-chromosome related
sequence in stealth virus-1 matches better to the human genome,
than to the genome of the African green monkey. The possibility
exists, therefore, of the integration of monkey-derived genetic
sequences into the human genome. This intriguing topic will be
discussed in a subsequent article.
SCMV-derived stealth adapted viruses have presumably
entered into the human population as a consequence of using
SCMV infected African green monkeys to produce live polio virus
vaccines [21,22]. Earlier polio virus vaccines were produced in
cytomegalovirus infected rhesus monkeys. DNA from Rhesus
monkey cytomegalovirus (RhCMV) is present in some earlier
polio virus vaccines, including the CHAT vaccine, which was
experimentally tested in African chimpanzees [23]. This is
consistent with the report by Dr. Albert Sabin that the CHAT vaccine
had a contaminating cytopathic virus [24]. Indeed, it is likely that
stealth adapted RhCMV was involved in the transformation of
simian immunodeficiency virus (SIV) to human immunodeficiency
virus (HIV) [25].
A reasonable model for the incorporation of cellular sequences
into the virus genome is the hybridization of a fragment from the
virus genome with a molecule of single stranded cellular RNA. This
could lead to RNA or DNA synthesis of the complimentary sequence.
If a different virus fragment then bound to the newly synthesized
nucleic acid, it could essentially mimic the PCR within the cell.
What is required, therefore, is a degree of genetic complementary
between a fragment of the virus genome with a sequence in cellular
RNA, together with an effective polymerase enzyme. Various
cellular polymerases, including endogenous reverse transcriptase
enzymes [26], can potentially be involved in the replication process.
The polymerases of other viruses could similarly be involved.
This is relevant to the production of polio vaccine virus in SCMV
infected African green monkey kidney cells since the polio virus
would be a source of RNA polymerase [27]. Although, the cellular
gene incorporation process for stealth virus-1 may have begun in
African green monkeys, there may have been subsequent switching
or exchanging of the incorporated primate cellular sequences with
the related human cellular sequences. This type of exchange would
evolve over time once humans became infected. The is consistent
with the relatively close relationship of the PCR amplified sequence
from the human X chromosome with the counterpart sequences
in the genomes of primates. Stealth virus-1 induces a severe acute
illness in cats [28]. To test the possibility of genetic exchange, virus
passaged in cats could be periodically examined for an exchange of
the human sequences with feline sequences. Similar studies can be
conducted in the many species of animal cells in which the stealth
adapted virus can be cultured [1].
The process by which cellular sequences have become part
of the virus replication process can seemingly also apply to the
incorporation of bacterial sequences. There is no evidence that the
stealth adapted cultures were infected with any bacteria. Specifically,
on several occasions, subcultures were maintained for extended
periods in antibiotic free media. Regular microscopy, including the
high-power examination of hematoxylin and eosin (H&E) stained
cells, showed no bacteria, nor did electron microscopic examination
of the infected cells. Rather it appears that the incorporation of
bacteria-derived sequences was a prior event occurring during or
shortly after the stealth adaptation process. The terms “viteria”
and “vifungus” were suggested to describe eukaryotic viruses with incorporated bacterial or fungal sequences, respectively
[10,29]. Of interest are the potential locations at which virus and
bacterial sequences would have the opportunity to interact. For
intracellular bacteria, such as mycoplasma, borrelia, and brucella, it
could be within a dually infected eukaryotic cell. The incorporation
of ochrobactrum sequences into a stealth adapted virus probably
requires a different explanation. Conventional eukaryotic viruses
do not ordinarily grow in bacteria. The genes coding for the growthrestrictive
elements may, however, be deleted during the stealth
adaptation process. Stealth virus-1 is cytopathic for cells of multiple
species, including insects. There is also evidence of stealth adapted
viruses affecting bacteria. For example, atypical bacteria were
cultured from the feces of the stealth virus-1 infected CFS patient.
Moreover, transmissible cytopathic activity was subsequently
retrieved from the atypical bacterial colonies (unpublished).
Ochrobactrum bacteria are generally regarded as being soil-based,
although Ochrobactrum anthropi can occasionally infect humans
[30]. Humans can, however, be exposed to these bacteria in
consumed food [31]. These bacteria may also potentially reside in
the gut microbiota. Unlike the non-coding cellular sequences, many
of the identified bacteria sequences have open reading frames and
would presumably lead to the formation of proteins. The bacterial
proteins, including enzymes, may potentially contribute to the
virus replicative process and to the induced cytopathology. The
proteins could also potentially function as a makeshift capsid for
the intercellular transfer of replicated nucleic acids. To do so, the
bacterial proteins would presumably need to self-assemble into
cage-like structures, which are able to enclose strands of nucleic
acids. Although speculative as to its relevance, it is interesting to
relate the incorporation of bacterial sequences to a self-healing
process, which occurs during the culturing of stealth adapted
viruses. The healing process is associated with the production of
self-assembling aliphatic and aromatic chemical compounds. The
assembled materials are referred to as alternative cellular energy
(ACE) pigments [32]. These materials can attract an external force
called KELEA (Kinetic Energy Limiting Electrostatic Attraction),
which drives the ACE pathway [33-37]. Enhancing the ACE pathway
can lead to the suppression of the virus induced CPE [32]. It would
not be surprising, therefore, if some of the bacterial gene-coded
proteins were to be subsequently identified as contributing to
the formation of ACE pigments. These pigments could potentially
prolong the infection process. Bacterial proteins might also be
expected to lead to intracellular protein aggregates and to trigger
the unfolded protein response [38]. This response can also
potentially lead to a delay in virus-induced cell death, thereby,
allowing for a more prolonged symbiotic relationship. A diverse
array of intracellular and extracellular aggregates can be seen by
electron microscopy in stealth adapted virus-infected cultured cells
and in the biopsied tissues from infected individuals.
The relocation of genetic sequences from their normal cellular
or bacteria origin to a virus can be viewed as a passive hijacking
of the sequences by the virus or as a loss of the cell’s or the
bacteria’s capacity to restrain some of their own genetic sequences
from deserting and moving elsewhere. In this context and to help
in conveying an understanding of the process, the relocating
sequences are being referred to as renegade sequences. The
capturing of cellular sequences by viruses is not a new phenomenon
[39]. Indeed, even regular SCMV encodes five copies of a G-protein
coding gene, which was presumably originally cellular in origin
[40]. What is different with regards to the present findings is
the concept that the incorporated genes could potentially come
to comprise an essentially autonomous, replicating, cytopathic,
renegade virus. In other words, once the replicative process
involving cellular sequences is underway, there may be no longer
a need for remaining sequences of the initiating stealth adapted
virus. In this regard, stealth virus-1 may be relatively early in its
evolution with its retention of extensive SCMV-related sequences.
Viruses are known to engage with noncoding RNA elements
within the cell. The interactions can either be advantageous
or inhibitory to the growth of the virus, depending on what
particular RNA element is being affected [41-46]. These previous
reports describe the cell and the viruses as separate and discrete,
although interactive, entities. A fundamentally different concept
is the switching of certain noncoding DNA or RNA elements from
primarily belonging to the cell to becoming part of the replicating
and transmissible virus genome. Since noncoding sequences
have specificity in their activities [47,48], the type or types of
incorporated sequences could have a bearing on the cytotoxic or
tumorigenic effects of the modified (renegade) virus.
Major substitutions of renegade sequences for the original
virus sequences can have important diagnostic implications.
On the one hand, the presence of bacteria-derived sequences in
stealth adapted viruses can potentially lead to mistaking a virus
infection for a bacterial infection. Examples where this error is
probably occurring include i) Attributing so called chronic Lyme
disease to an active infection by Borrelia burgdorferi [49]. Indeed,
there is good unpublished evidence from performing stealth virus
cultures in many patients diagnosed with chronic Lyme disease,
that the individuals are virally infected. It remains to be shown
that the viruses contain borrelia-related genetic sequences. ii)
Also mistaking Morgellons skin disease for an infection with
Borrelia, Agrobacteria, or other parasites [49-51]. There have
been no examples of actually culturing bacteria from these
patients. Many of those tested, however, have had positive stealth
virus cultures. iii) Linking CFS with mycoplasma [52,53] or with
brucella infection. An interesting personal communication from a
mycoplasma researcher was that the mycoplasma sequences he
was identifying were actually slightly different from those of the
known mycoplasma species. Some CFS patients have been tested
for brucella antibodies and found to be positive. iv) Defining
PANDAS (Pediatric Autoimmune Neuropsychiatric Disorders
Associated with Streptococcal Infections) as a bacteria-induced
disease [54,55]. Again, on close analysis there is often a discrepancy
between the anti-streptolysin O and anti-DNase B antibody testing
in patients diagnosed with PANDAS (personal observation). v) Associating bacteria, such as Porphyromonas gingivalis [56,57] or
chlamydia [58-60] with Alzheimer disease and with rheumatoid
arthritis [61,62]. The prospect of viteria infections may help in
reorienting the thinking about these associations. An even bigger
issue is the many illnesses in which a virus infection is somewhat
suspected, yet the regular molecular and serological screenings
for known viruses yield negative results. There is typically no
accompanying inflammation associated with these illnesses.
Prominent examples of these illnesses include CFS, fibromyalgia,
autism, multiple sclerosis, amyotrophic lateral sclerosis, bipolar
depression, schizophrenia, and Alzheimer disease. Some of the
controversies related to viruses causing such illnesses are covered
in the references [63-78]. Glioblastoma is a further example of a
disease with suggestive evidence of an underlying virus infection
[79-81]. A few cases tested by stealth virus culture did give positive
results. Until disproven, many persisting illnesses, including
cancers, should be studied for evidence of a possible contribution
by an ongoing infection with stealth adapted/renegade viruses.
The brain is particularly susceptible to symptomatic illness due
to stealth adapted viruses [76-78]. This is because of the spatial
distribution of the various aspects of brain functioning. It is unlike
other organs in which limited localized cell damage can be easily
compensated by overactivity elsewhere in the same organ. There
has been an overall increase in mental illness, and this may well be
due to an epidemic of stealth adapted virus infections.
Stealth adapted/renegade viruses can best be detected using
virus cultures that consider the production of ACE pigments. The
presence of disease-causing viruses can be further confirmed
by inoculation of the cultured material into animals [28]. The
detection of stealth adapted induced illnesses is important in
disease prevention and is relevant to the safety of the nation’s
blood supply. It is also relevant to the risk of vaccine triggering of
a tissue damaging immune response against some of the normally
non-immunogenic components in these viruses. Fortunately, the
body’s ACE pathway has the capacity to suppress stealth adapted
viruses. Clinical studies are needed to evaluate the effectiveness of
the various means of enhancing the ACE pathway in the therapy of
stealth adapted/renegade virus infections [82-90].
Summary
The process of stealth adaptation of viruses has been extended
beyond the deletion or mutation of the genes coding for the relatively
few components normally targeted by the cellular immune system.
Stealth adaptation can also involve the incorporation of added
sequences, which can be cellular and microbial in origin. The
incorporated sequences can lead to the further elimination of the
originating virus sequences, with the reformed virus retaining the
capacity for replication and transmission and, thereby, the ability
to cause diseases. The switching of cellular and bacterial sequences
into the virus world is likened to the actions of a renegade. The term
renegade is, therefore, being introduced as an added description of
stealth adapted viruses. Conventional serological and molecular
assays for viruses are inadequate as a screening method for
renegade viruses. Many chronic illnesses, including some cancers,
are likely caused by renegade viruses. They are best treated by
enhancing the alternative cellular energy (ACE) pathway.
Acknowledgement
I am grateful to the individuals who were unswayed when
learning that senior Public Health officials had declared that stealth
adapted viruses do not exist and that the clinical testing for the
viruses had put the Nation’s health in Immediate Jeopardy. This
article with a slight change in the Title has also been published
in the Journal of Human Virology and Retrovirology. Duplication
is intended and agreed upon to help reach as wide a readership
as possible given the significance of infectious monkey-derived
cellular sequences being transmitted to humans.
References
- Martin WJ,
Zeng LC, Ahmed K, Roy M (1994) Cytomegalovirus-related sequence in an
atypical cytopathic virus repeatedly isolated from a patient with
chronic fatigue syndrome.The American Journal of Pathology 145(2):
440-451.
- Ehrlich GD, Glaser JB, LaVigne K, Quan D, Mildvan D, et
al. (1989) Prevalence of human T-cell leukemia lymphoma virus (HTLV)
type II infection among high-risk individuals: Type-specific
identification of HTLVs by polymerase chain reaction. Blood 74: 1658.
- Johnson M,
Zaretskaya I, Raytselis Y,Merezhuk Y, McGinnis S, et al. (2008) NCBI
BLAST: a better web interface. Nucleic Acids Research 36: 5-9.
- Martin
WJ, Ahmed KN, Zeng LC, Olsen JC, Seward JG, et al. (1995) African green
monkey origin of the atypical cytopathic “stealth virus” isolated from a
patient with chronic fatigue syndrome.Clinical and Diagnostic Virology
4(1): 93-103.
- Martin WJ
(1999) Stealth adaptation of an African green monkey simian
cytomegalovirus.Experimental and Molecular Pathology 66(1): 3-7.
- Martin WJ (2014) Stealth adaptation of viruses: Review of earlier
studies and updated molecular analysis of a stealth adapted African
green monkey simian cytomegalovirus. In Stealth Adapted Viruses;
Alternative Cellular energy pp. 3-30.
- Martin WJ (2014) Stealth adaptation of viruses: Review and updated
molecular analysis on a stealth adapted African green monkey simian
cytomegalovirus (SCMV). Journal of Human Virology & Retrovirology
1(4): 00020.
- Martin WJ (1998) Cellular sequences in stealth viruses.Pathobiology 66: 53-58.
- Martin WJ
(1999) Melanoma growth stimulatory activity (MGSA/GRO-α) chemokine genes
incorporated into an African green monkey simian
cytomegalovirus-derived stealth virus.Experimental and Molecular
Pathology. 66(1): 15-18.
- Martin WJ
(1999) Bacteria-related sequences in a simian cytomegalovirus-derived
stealth virus culture. Experimental and Molecular Pathology. 66(1):
8-14.
- JohannsonKE, PetterssonB (2002)Taxonomy of mollicutes. Molecular Biology and Pathogenicity of Mycoplasmas,
- Calderon CS,
Wigger G, Wunderlin C, Schmidheini T, Frey J, et al. (2009) The
Mycoplasma conjunctivae genome sequencing, annotation and analysis. BMC
Bioinformatics. 10(6): S7.
- Lo SC, Hayes
MM, Tully JG, Wang RY, Kotani H, et al. (1992)Mycoplasma penetrans sp.
nov., from the urogenital tract of patients with AIDS. Int J Syst
Bacteriol 42(3): 357-364.
- Paulsen IT,
Seshadri R, Nelson KE, Eisen JA, Heidelberg JF, et al. (2002) The
Brucella suis genome reveals fundamental similarities between animal and
plant pathogens and symbionts. Proceedings of the National Academy of
Sciences 99(20): 13148-13153.
- Scholz HC,
Dahouk S, Tomaso H, Neubauer H, Witte A, et al. (2008) Genetic diversity
and phylogenetic relationships of bacteria belonging to the Ochrobactrum Brucella group by recA and 16S rRNA gene-based comparative sequence analysis. Syst Appl Microbiol 31(1): 1-16.
- Krzyżanowska DM, Maciąg T, Ossowicki A, Rajewska M, Kaczyński Z, et al. (2019) Ochrobactrum quorumnocens sp nov
a quorum quenching bacterium from the potato rhizosphere, and
comparative genome analysis with related type strains. PLoS ONE 14(1):
e0210874.
- Huber B, Scholz HC, Kämpfer P, Falsen E, Langer S, et al. (2016) Ochrobactrum pituitosum sp nov isolated from an industrial environment. Int J Syst Evol Microbiol 60(2): 321-326.
- Kampfer P (2007) Ochrobactrum haematophilum sp nov and Ochrobactrum pseudogrignonense sp nov isolated from human clinical specimens. International Journal of Systematic and Evolutionary Microbiology 57(11): 2513-2518.
- Martin WJ (1996) Genetic instability and fragmentation of a stealth viral genome.Pathobiology 64(1): 9-17.
- Martin WJ
(1996) Simian cytomegalovirus-related stealth virus isolated from the
cerebrospinal fluid of a patient with bipolar psychosis and acute
encephalopathy. Pathobiology 64(2): 64-66.
- Sierra HA,
Krause M, Philip R (2002) Live oral poliovirus vaccines and simian
cytomegalovirus. Journal of the International Association of Biological
Standardization 30(3): 167-174.
- Baylis SA,
Shah N, Jenkins A, Berry NJ, Minor PD (2003) Simian cytomegalovirus and
contamination of oral poliovirus vaccines. Biologicals 31(1): 63-73.
- Berry N,
Davis C, Jenkins, A, Wood D, Minor P, et al. (2001) Vaccine safety:
Analysis of oral polio vaccine CHAT stocks. Nature 410(6832): 1046-1047.
- Sabin AB (1959) Present position on immunizing against poliomyelitis with live virus vaccines. Brit Med J 1(5123): 663-80.
- Martin WJ (2015) Chimpanzees inoculated with cytomegalovirus
contaminated polio vaccines may explain origin of HIV-1. Journal of
Human Virology & Retrovirology 2(2): 00035.
- Abraham GN, Khan AS (1990) Human endogenous retroviruses and immune disease. Clin Immunol Immunopathol 56(1): 1-8.
- Barton DJ,
Flanegan JB (1993) Coupled translation and replication of poliovirus RNA
in vitro: synthesis of functional 3D polymerase and infectious
virus. The Journal of Virology 67(2): 822-82231.
- Martin WJ, Glass
RT (1995) Acute encephalopathy induced in cats with a stealth virus
isolated from a patient with chronic fatigue syndrome. Pathobiology 63:
115‑118.
- Martin WJ (2005) Alternative cellular energy pigments mistaken for parasitic skin infestations. Exp Mol Path 78(3): 212-214.
- Holmes B, Popoff M, Kiredjian M, Kersters K (1988) Ochrobactrum
anthropigen nov sp nov from human clinical specimens and previously
known as group Vd. Int J Syst Bacteriol 38: 406-416.
- Jaykus LA, Wang HH, Schlesinger LS (2009) Food borne microbes: shaping the host ecosystem. ASM Press, USA.
- Martin WJ (2003) Stealth virus culture pigments: a potential source of cellular energy. Exp Mol Path 74: 210-223.
- Martin WJ (2015) KELEA: A natural energy that seemingly reduces
intermolecular hydrogen bonding in water and other liquids. Open Journal
of Biophysics 5(3): 69-79.
- Martin WJ (2015) Interacting light paths attract KELEA (kinetic
energy limiting electrostatic attraction) and can lead to the activation
of water. Open J Biophysics 5(4): 115-121.
- Martin WJ (2015) Interacting electric fields attract KELEA (kinetic
energy limiting electrostatic attraction) and can lead to the activation
of water. International J Complementary & Alternative Medicine
1(6): 00034.
- Martin WJ (2016) KELEA (kinetic energy limiting electrostatic
attraction) may add to the measured weight of an object. J Modern
Physics 7(6): 461-472.
- Martin WJ (2016) KELEA cosmic rays cloud formation and
electromagnetic radiation: Electropollution as a possible explanation
for climate change. Atmospheric and Climate Sciences. 6(2): 174-179.
- Cao SS, Kaufman RJ (2012) Unfolded protein response. Current Biology 22(16): R622-R626.
- Dagna L (2007) Virus-encoded chemokines, chemokine receptors and
chemokine-binding proteins: new paradigms for future therapy. Future
Virol 2(4): 353-368.
- Sahagun RA,
Sierra HA, Krause P, Murphy PM (2004) Simian cytomegalovirus encodes
five rapidly evolving chemokine receptor homologues. Virus Genes 28(1):
71-83.
- Wang P, Xu J,
Wang Y, Cao X (2017) An interferon-independent lncRNA promotes viral
replication by modulating cellular metabolism. Science 358(6366):
1051-1055.
- Goodwin CM,
Xu S, Munger J (2015) Stealing the keys to the kitchen: viral
manipulation of the host cell metabolic network. Trends Microbiol
23(12): 789-798.
- Mesquita I, Estaquier J (2018) Viral manipulation of the host
metabolic network. Metabolic Interaction in Infection Experientia
Supplementum 109: 899-989.
- Powdrill MH,
Desrochers GF, Singaravelu R, Pezacki JP (2016) The role of microRNAs in
metabolic interactions between viruses and their hosts. Curr Opin Virol
19: 71-76.
- Ghosh Z,
Mallick B, Chakrabarti J (2009) Cellular versus viral microRNAs in
host-virus interaction. Nucleic Acids Res 37(4): 1035-1048.
- Fiorucci G,
Chiantore MV, Mangino G, Romeo G (2015) MicroRNAs in virus-induced
tumorigenesis and IFN system. Cytokine Cell Growth Rev 26(2): 183-194.
- Mohebbi A,
Tahamtan A, Eskandarian S, Askari FS, Shafaei M, et al. (2018) Viruses
and long non-coding RNAs: implicating an evolutionary conserved
region. Virusdisease 29(4): 478-485.
- Slack FJ, Chinnaiyan (2019) The role of noncoding RNA in oncology. Cell 177(5): 1033-1055.
- Middelveen
MJ, Bandoski C, Burke J (2015) Exploring the association between
Morgellons disease and Lyme disease: identification of Borrelia
burgdorferi in Morgellons disease patients. BMC dermatology 15(1): 1-1.
- Middelveen
MJ Stricker RB (2016) Morgellons disease: a filamentous borrelial
dermatitis. International Journal of General Medicine 9: 349-354.
- Middelveen
MJ, Filush KR, Bandoski C, Kasliwala RS, Melillo A, et al. (2019)
Mixedborrelia burgdorferi and helicobacter pylori biofilms in morgellons
disease dermatological specimens. Healthcare (Basel) 7(2): 70.
- Nijs Jo, Nicolson GL, De Becker P, Coomans D, De Meirleir K (2002) High prevalence of Mycoplasma infections among European chronic fatigue syndrome patients. Examination of four Mycoplasma species in blood of chronic fatigue syndrome patients. FEMS Immunology and Medical Microbiology 34(3): 209-214.
- Endresen G
(2003) Mycoplasma blood infection in chronic fatigue and fibromyalgia
syndromes. Rheumatology International 23(5): 211-215.
- Swedo SE,
Leonard HL, Garvey M, Mittleman B, Allen AJ, et al. (1998) Pediatric
autoimmune neuropsychiatric disorders associated with streptococcal
infections: clinical description of the first 50 cases. Am J Psychiatry
155(2): 264-271.
- March JS
(2004) Pediatric autoimmune neuropsychiatric disorders associated with
streptococcal infection (PANDAS): implications for clinical practice.
Arch Pediatr Adolesc Med 158(9): 927-929.
- Dominy SS, Lynch C, Ermini F (2019) Porphyromonas gingivalis
in Alzheimer’s disease brains: Evidence for disease causation and
treatment with small-molecule inhibitors. Science advances. 5(1): 3333.
- Singhrao SK, Harding A, Poole S, Kesavalu, L, Stjohn C (2015) Porphyromonas gingivalis periodontal infection and its putative links with Alzheimer’s disease. Mediators of Inflammation
- Shima K,
Kuhlenbäumer G, Rupp J (2010) Chlamydia pneumoniae infection and
Alzheimer’s disease: a connection to remember? Medical Microbiology and
Immunology 199(4): 283-289.
- Appelt DM,
Howanski RJ, Hallock LR, Hammond CJ, Scott LC et al. (2010)
Immunohistological detection of Chlamydia pneumoniae in the Alzheimer’s
disease brain. BMC Neuroscience 11(1): 121.
- Gérard HC.
Dreses WU, Wildt KS (2006) Chlamydophila (Chlamydia) pneumoniae in the
Alzheimer’s brain. FEMS Immunology & Medical Microbiology 48(3):
355-366.
- Loyola RJP,
Martinez MRE, Abud MC, Patiño MN, Seymour GJ (2010) Rheumatoid arthritis
and the role of oral bacteria.Journal of Oral Microbiology 2(1): 1-8.
- Andersen I, Olesen HP (1990) Anaerobic bacteria in rheumatoid arthritis.Annals of the Rheumatic Diseases 49(7): 568.
- Kendell R (1991) Chronic fatigue, viruses, and depression.Lancet 337: 160-162.
- Branco JC, Tavares V, Abreu I, Humbel RL (1994) Viral infection in fibromyalgia. Acta Med Port 7(6): 337-341.
- Sprott H (2011) Is fibromyalgia a viral disease? Z Rheumatol 70(8): 637-638.
- Virtanen JO, Jacobson S (2016) Viruses and Multiple Sclerosis. CNS Neurol Disord Drug Targets 11(5): 528-544.
- Kakalacheva
K, Comabella M (2010) Epstein barr virus and multiple sclerosis:
causation or association? Future Microbiology 5(11): 1617-1619.
- Yolken RH, Torrey EF (1995) Viruses, schizophrenia, and bipolar disorder. Clin Microbiol Rev 8(1): 131-145.
- Libbey JE, Sweeten TL, McMahon WM, Fujinami RS (2005) Autistic disorder and viral infections. J Neurovirol 11(1): 1-10.
- Martin WJ (1995) Stealth virus isolated from an autistic child. J Autism Dev Disord 25(2): 223-224.
- Weiner PL, Stohlman,AS, Davis, LR (1980) Attempts to demonstrate
virus in amyotrophic lateral sclerosis. Neurology 30(12): 1319-1322.
- Corcia P,
Giraud P, Guennoc AM, Toffol B, Autret A (2003) Acute motor axonal
neuropathy, enterovirus and amyotrophic lateral sclerosis: can there be a
link? Revue Neurologique 159(1): 80-82.
- Xue Y, Chao
FR, Cashman N, Luo H (2018) Enteroviral infection: The forgotten aink to
amyotrophic lateral sclerosis? Frontiers in Molecular Neuroscience 11:
63.
- Torrey EF (1988) Stalking the schizovirus. Schizophrenia bulletin 14(2): 223-229.
- Libikova H (1983) Schizophrenia and viruses: principles of etiologic studies. Advances in Biological Psychiatry 12: 20-51.
- Peacrce DB (2003) Can a virus cause schizophrenia? KUWER Academic Publishers, London, UK.
- Crow TJ (1978) Viral causes of psychiatric disease. Postgrad Med J 54: 763-767.
- Hare EH (1979) Schizophrenia as an infectious disease. British Journal of Psychiatry 135: 468-470.
- Cosset E,
Petty TJ, Dutoit V, Cordey S, Padioleau I, et al. (2014) Comprehensive
metagenomic analysis of glioblastoma reveals absence of known virus
despite antiviral‐like type I interferon gene response. International
Journal of Cancer 135(6): 1381-1389.
- Cobbs
CS, Harkins L, Samanta M (2002) Human cytomegalovirus infection and
expression in human malignant glioma. Cancer Res 62(12): 3347-3350.
- Scheurer
ME, Bondy ML, Aldape K (2008) Detection of human cytomegalovirus in
different histological types of gliomas. Acta Neuropathol 116(1): 79-86.
- Martin WJ
(1996) Severe stealth virus encephalopathy following chronic fatigue
syndrome‑like illness: Clinical and histopathological features.
Pathobiology 64: 1‑8.
- Martin WJ (2015) Stealth adapted viruses -possible drivers of major
neuropsychiatric illnesses including Alzheimer’s disease. J Neurol
Stroke 2(3): 00057.
- Martin WJ (2015) Stealth adapted viruses: a bridge between molecular
virology and clinical psychiatry. Open Journal of Psychiatry5(4):
311-319.
- Martin WJ (2015) Therapeutic potential of KELEA activated water.
International Journal of Complementary & Alternative Medicine 1(1):
00001.
- Martin WJ (2017) Cancer is treatable via the alternative cellular energy (ACE) pathway. J Cancer Therapy 8(13): 1279-1290.
- Martin WJ (2016) The ACE pathway in comparison to the immune system
in the defense against infectious diseases. J Human Virology &
Retrovirology 3(5): 00124.
- Martin WJ (2016) Insufficiency of cellular energy (ICE) the basis
for many illnesses potentially correctable using KELEA activated water.
International J Complementary & Alternative Medicine 4(1): 00106.
- Martin WJ (2015) Stealth adaptation of viruses: implications for
therapy and for potential toxicity of vaccines. JOJ Immuno Virol 1:
555551.
- Martin WJ (2020) Enhancing the alternative cellular energy (ACE)
pathway with KELEA activated water as therapy for infectious diseases.
Infectious Disorders Drug Targets.